Prof. Alexandre Tkatchenko of the University of Luxembourg addresses one of the greatest mysteries in physics: dark energy.
What lies between the stars? And why is the Universe ‘growing’ faster and faster?
Physicists are currently coming up with the same probable answer to these questions: dark energy. This hypothetical entity, which accounts for two-thirds of all the energy in the Universe, is thought to be responsible for its accelerated expansion. However, we don’t really know what it is.
Defining this energy and calculating its precise value could help bring together two major schools of thought: quantum field theory (QFT) and the general theory of relativity developed by Albert Einstein. The only problem is that, until now, QFT calculations have been far from consistent with the observations made by our telescopes. This discrepancy has haunted physicists for more than a century.
In Quantum Field Theory (QFT), elementary particles are described not as corpuscles (little balls) but as oscillations (vibrational modes) in fundamental fields that exist at every point in space and time.
A quantum field is not easy to visualise. It is an abstract mathematical object designed to calculate probabilities. Nevertheless, it could be described as a canvas stretched across space, with each point having a precise value. In this context, “particles” do not exist as such. They are in fact representations of a disturbance propagating within the web. That’s why quantum physics call them “wave packets”.
QFT describes a multitude of fields: electric, magnetic, photonic…
In physics, QFT is the most precise theory of all, but it presents some major challenges. Hardly applicable to the macroscopic world, it still can’t describe gravitation. What’s more, the mathematical computations involved in QFT are extraordinarily complex, which means that many things can’t be calculated.
That may well change in the future, thanks to the work of Prof. Tkatchenko’s team from the Department of Physics and Materials at the University of Luxembourg. In a pioneering publication, published in January 2023 in the prestigious journal Physical Review Letters, they set out a completely new definition of this mysterious dark energy.
To help us understand, we asked him some questions about his findings.
What is dark energy, and what problems does it raise?
Dark energy is everywhere, but most of it lies between the stars. It could account for up to 70% of the energy in our Universe.
‟ Dark energy determines the dynamical evolution of the entire universe. You can’t understand the universe without explaining it”
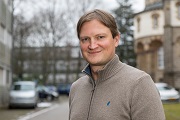
Full professor in Theoretical Condensed Matter Physics
But if it is everywhere, why do we know so little about it?
“In our daily lives, its influence is not felt. It is within our tables and the rest of matter, but we have no way of “feeling it” with available instruments — all the other effects on earth are much stronger. So, at first sight, it seems of limited interest to us. But for the universe, it is the most important phenomenon: it is the force that opposes gravitation” (the attractive force that acts between massive objects, such as galaxies).
You could think of it as two hands pulling on the fabric of the universe: one – gravitation – to roll it into a ball, the second – dark energy – to stretch it. The latter seems to be winning the battle, preventing the Universe from collapsing and even accelerating its expansion.
The problem is that, until now, when physicists tried to pin down its exact value – via the cosmological constant – using quantum field theory, the result was between 60 and 120 orders of magnitude greater than that measured by our telescopes! This made it impossible to represent what it is, blocking our understanding of the Universe.
However, thanks to the new approach developed by Prof. Tkatchenko’s team, this discrepancy could be eventually resolved, making it possible to calculate a cosmological constant that is identical in quantum physics and astrophysics.
So, what does this mean? Let’s first define what this cosmological constant is, and how it relates to dark energy.
What is the cosmological constant, and how does it relate to dark energy?
Prof. Tkatchenko explains: “Our telescopes (Hubble, Planck and Webb) take measurements in the Universe, and more specifically in the vacuum. This data is used to determine certain parameters of space and time. One of these parameters is the so-called cosmological constant, which has been known for 100 years thanks to Einstein’s equations. This constant essentially defines the energy density of the vacuum field, or what we commonly call dark energy”.
The cosmological constant was originally added by Einstein to his equations in 1917 to help maintain his belief that the universe is static, i.e., unchanging in time.
A few years later, American astronomer Edwin Hubble discovered that galaxies were moving away from each other, as in a stretched-out canvas, showing that the Universe is far from static.
From then on, the cosmological constant posed quite a problem for Einstein, so much so that he reportedly called it the biggest mistake of his life. He ended up setting it to zero in his equations, thus creating the Einstein-De Sitter model of space-time in 1931… But this was before new observations were made by telescopes in the 90s, showing an acceleration in the expansion of the Universe! And this acceleration can indeed be described by a positive cosmological constant, the exact value of which has yet to be determined.
Despite himself, Einstein was proven right once again.
But how dense can energy be in the Void?
“Actually, it is a truly minuscule energy density at every point in space,” notes Tkatchenko. The energy density of matter (the table, the earth, etc.) is much higher than the energy density of vacuum. But the latter constitutes an enormous percentage of the total energy density of the Universe: quite simply because the Universe is rather empty! There isn’t much matter, and there’s an enormous amount of space.”
What is the problem with the cosmological constant?
Quantum field theory predicts an enormous vacuum energy density (around 10^114 J/m3 or, in terms of mass, 10^95 g/cm3 if the Planck length of 10-35 m is used). But if this were the case, it would tear the universe apart. Astronomical data, on the other hand, indicate that such a cosmological constant (expressed in mass) must be 10^-29 g/cm3, which represents a discrepancy of 60 to 120 orders of magnitude depending on the method of calculation!
This staggeringly colossal mismatch is sometimes known as the “vacuum catastrophe” and represents one of the greatest problems in physics today.
Solving it would enable quantum field theory to finally catch up with general relativity, a veritable revolution in science, from the infinitely small to the infinitely large.
How does Prof. Tkatchenko’s team solve the problem?
Let’s start by explaining their findings.
The vacuum is not empty (that would be too simple!). The “vacuum”, i.e., space devoid of matter, has energy and can be considered as a quantum field.
“Quantum fields have transient fluctuations of particle-antiparticle pairs, or ‘virtual excitations’,” explains Prof. Tkatchenko.
We could think of these as spontaneous appearances of particles with an infinitesimal life span, but capable, during their brief existence, of interacting with each other like any other particles. Like ghosts appearing in an empty room, exchanging a few words, and disappearing in the blink of an eye. This is why the vacuum is not empty.
These “virtual excitations” form so many tiny, short-lived dipoles. In other words, our ghosts play tennis before they vanish.
In physics, a dipole can be either magnetic or electric. The most basic electric dipole is formed by two equal but opposite electrical charges. This is the case of the virtual electron-positron pairs that form in a vacuum. These dipoles then interact with the electromagnetic field, making the vacuum behave like a polarised medium.
The polarisation of a dipole is its response to an applied electric field. In any given system, the separation of positive and negative electric charges is a measure of its overall polarity.
Prof. Tkatchenko explains: “The interactions of these dipoles lead to a vacuum polarisation density. This is what we call ‘vacuum self-interaction’. Our main contribution is to define this polarisation”.
This is the innovative – and particularly elegant – idea behind their research. Because this intrinsic and finite polarisation of the vacuum can be measured, calculated, and integrated into the pre-existing equations of physics.
This new way of understanding the void is conceptually efficient. It introduces no novel fields, particles, or constants. It is born of a pre-existing framework, and in this respect, it is particularly appealing.
Such conceptual elegance is known as Ockham’s razor principle, also known as the “law of parsimony”, which states that the simplest theory is the one most likely to be right. Ockham’s razor continues to guide scientific thinking and offers many advantages, not least in the extraordinarily complex field of quantum mechanics, where any new entity brings with it a host of fresh, unsolvable problems.
In fact, it was the principle of parsimony that made Einstein so annoyed with the cosmological constant!
“We have defined this polarisation in previous work, based on models we have for atoms,” explains Tkatchenko. “Usually, we use quantum mechanics to describe physical phenomena, but QFT doesn’t really help us to understand the nature of reality. We have gone in the opposite direction, taking what we know about the molecular world and applying it to quantum fields”.
One of the most fascinating debates in physics is the one that took place between two strong-minded friends: Niels Bohr, one of the founders of quantum mechanics, and Albert Einstein, the father of general relativity. So, what was at stake? Nothing less than reality as we perceive it, or rather, reality as it is – independently of our perception.
Following ontological question famously illustrates the problem: does the tree in the forest make noise when it falls, if no one is there to hear it? In other words: does reality as we imagine it exist independently of us, and if so, what does it look like?
For Einstein, a falling tree remains a tree, with a well-defined colour, shape, and speed of fall, whether we’re there or not. But for Bohr and quantum physics, this is no longer the case. The unobserved tree is in all states at once: it falls and stands, its colour and shape are undefined. And what’s even more amazing: if we measure one of its parameters, such as its falling speed, then we can no longer know its colour, and vice versa.
What, then, becomes of the very concept of a tree? How do we represent it? Quantum mechanics, a spectacularly efficient theory (responsible, among other things, for major innovations such as lasers, transistors, geolocation clocks…), alas, provides no answer that is easy to visualise. It teaches us that we can predict without necessarily understanding.
Enough to drive one mad – and make us wonder!
The value thus obtained,” explains Prof. Tkatchenko, “is consistent with the observed value of the cosmological constant. More precisely, it lies between the two recent measurements of the cosmological constant Λ obtained by the Planck mission and the Hubble space telescope.”
Prof. Tkatchenko’s team has therefore succeeded in extracting a cosmological constant corresponding to the one observed in astrophysics, and not the one calculated in quantum field theory.
And the name of the publication? It is entitled “Casimir Self-Interaction Energy Density of Quantum Electrodynamic Fields”. Crystal clear!
The Casimir effect, also known as the Casimir force, is a (very weak) force arising from quantum fluctuations in vacuum (those pairs of ghosts that appear in an empty room). It owes its name to Dutch physicist Hendrik Casimir, who first described it in 1948.
The Casimir effect can be illustrated by what happens between two mirrors placed a few nanometres apart in vacuum. The closer they are to each other, the harder it is to pull them apart. This is possible because the mirrors modify the quantum fluctuations of vacuum: in simplified terms, the virtual photons that appear everywhere around them are numerically superior to those in the space between them, creating a positive force that brings the mirrors closer together.
When applied to astrophysics, the Casimir effect could be responsible for the origin of the universe: vacuum fluctuations are thought to have produced the primordial irregularities necessary for the formation of stars and galaxies.
The Casimir effect also has a direct impact on the nanotechnologies currently being developed (we can easily imagine how annoying it is to assemble tiny components that start sticking together in an awkward way…).
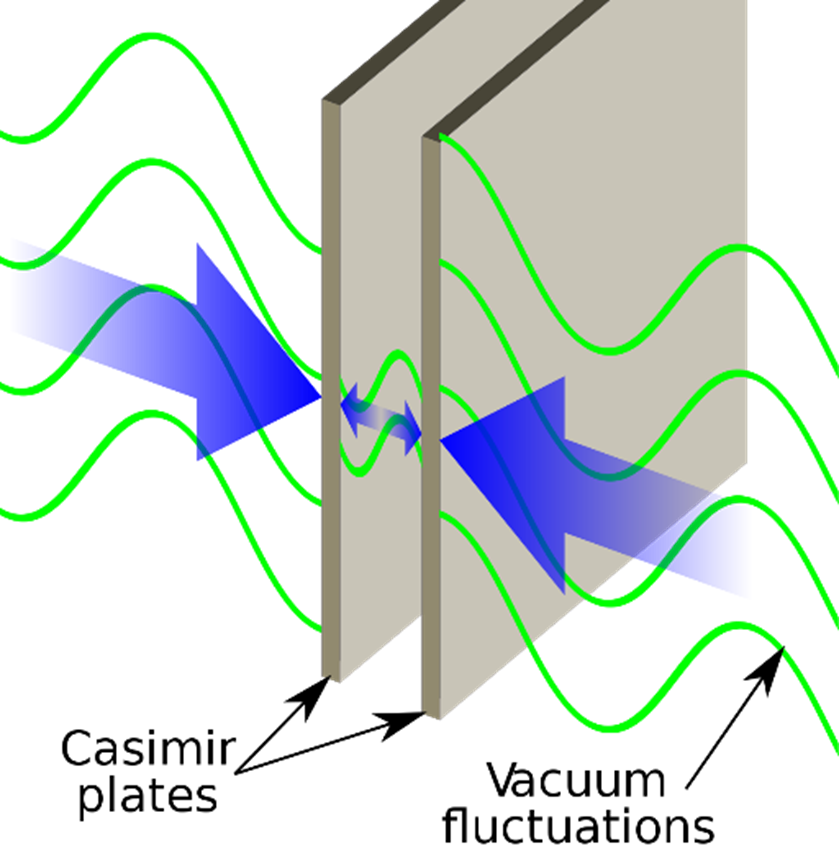
Source: Wikipedia Commons ; Author: Emok
Has this new approach already been put to the test?
As always in science, any new theory requires a rigorous testing phase to verify its validity. If the predictions it generates are confirmed by experiments, then it is valid until proven otherwise.
Tkatchenko says: “Our prediction is that there is an intrinsic polarisation of the vacuum field, even in the absence of matter. This is a falsifiable prediction, and we hope that future experiments will confirm it. In that case, it will be indisputable that the value we have found is the cosmological constant”.
On the other hand, fundamental quantum field theory could validate it by calculation. “Some experts in my group are currently working on this”, says Tkatchenko.
Let’s hope they succeed!
Apart from calculating the cosmological constant, what is the scope of this new approach and what possibilities does it present?
The possibilities are immense!
Indeed, if the approach taken by Prof. Tkatchenko and his team proves to be correct, it could integrate everything up to and including gravitation, paving the way for a grand unifying theory of general relativity and quantum mechanics. Such a theory would truly transform the world of physics.
To this day, the best theory to describe gravitation remains the theory of general relativity, formulated by Einstein in 1915 and published by the Royal Prussian Academy of Sciences. He was not the only one to elaborate it (Marcel Grossmann and David Hilbert are credited with some of the work). Their joint work truly transformed our understanding of the Universe.
An image often used to explain it is that of a stretched canvas (space-time) on which a marble is rolled. The ball’s mass deforms the cloth, creating the curvature we call “gravitation”, which deflects the ball’s trajectory. Strictly speaking, Gravitation is but the manifestation of this curvature.
General relativity encompasses and goes beyond Isaac Newton’s theory of gravitation (the famous falling apple), which is limited to low speeds and weak gravitational fields.
Indeed, gravitation has not yet been described by quantum mechanics, despite it being one of the four fundamental interactions of our universe (gravitational, electromagnetic, strong nuclear and weak nuclear). In the world of the infinitely small, the other three forces are extremely powerful, eclipsing the effect of gravitation to the point of making it inaccessible to calculation.
“We have already been able to obtain a derivation that seems to correspond to the gravitational force”, says Tkatchenko. “Integrating gravitation has never been done before”.
Some examples of practical applications?
Understanding quantum interactions enables us to put them to practical use in the macroscopic world.
Tkatchenko explains: “All interactions in nature are based on quantum mechanics: aggregation, protein-protein interaction, etc. But this theory is yet very difficult to apply to large systems. The equations are highly complex, and the objects it manipulates (wave functions) are almost impossible to visualise.”
The Theoretical Chemical Physics Group at the University of Luxembourg is working to extend the understanding of quantum mechanics to nanometric and larger systems (of thousands to millions of atoms) by merging chemistry, physics, biology, mathematics, and computer science.
Their multidisciplinary work extends from the atom to the cosmos, from the infinitely small to the infinitely large.
On this subject, Tkatchenko cites the example of polymorphism in molecular crystals. Many drugs are available in the form of tablets (crystals). Depending on the structure of these crystals, the active principle of the drug works – or not. “We know which interactions stabilise the functional forms of drugs. This is an example of something very practical. The methods developed by my team are already used routinely by the pharmaceutical industry”.
In the field of nanotechnology, their work could also have very practical applications: the previously mentioned Casimir force impacts the assembly of nano- and micro-materials and creates a considerable logistical problem.
A final word?
According to Prof. Tkatchenko, dark energy is luminous, since it comes from the interaction of photons with an electric field…
The next time our children look up at the sky and ask us what’s between the stars, we can give them this wonderful answer: luminous energy.
Interview originally published on www.science.lu.
Original article author: Diane Bertel